Simple super-resolution biological imaging
Microscopic imaging has traditionally been constrained by the diffraction limit, which for visible light restricts spatial resolution to several hundred nanometers. Surpassing this limit would significantly benefit several disciplines, such as medical and material sciences. We developed a simple, generic super-resolution imaging method that improves the resolution of optical imaging by a factor of 2 to 3. Our approach uses microscope coverslips composed of a monolayer of microspheres with high refractive index positioned in a transparent elastomer layer.
We fabricated several different microscope coverslips by spin coating barium titanate glass (BTG) microspheres (refractive index n∼1.9–2.1) in a polydimethylsilaxane (PDMS) layer whose thickness can be controlled by the spin speed and duration. When the slide is placed over the specimen, a magnified virtual image is formed by the microsphere and captured by the objective lens: see Figure 1(a,b). To demonstrate the principle, we used a commercial Blu-ray® disk (BD) as the imaging object, the structure of which consists of stripes 200nm wide separated by gaps 100nm wide. Conventional microscopy cannot resolve the structure of the BD; however, it can be resolved using one of our coverslips, as illustrated in Figure 1(c). Improving the spatial resolution by microsphere-assisted imaging acts by enhancing the effective numerical aperture (NA) of the system1–3 and the extraordinary focusing properties of microspheres,4–6 the so-called photonic nanojet effect.7 It should be noted that the focusing properties of our microsphere-embedded films can find applications in other areas such as nano-scale patterning, spectroscopy, and photovoltaics. (Reviews are available elsewhere.1)

We also investigated the feasibility of using our microsphere-assisted technique to image biological structures, such as cells2 and tissue sections.3 A limiting factor in predicting patients' response to radiation therapy is the sensitivity of their tumor cells to ionizing radiation. This sensitivity can be assessed by observing the repair of double strand breaks (DSB) in DNA, which can be detected via phosphorylated histone γ-H2AX, a highly specific and sensitive molecular marker. Cells are exposed to radiation, and the number of γ-H2AX foci induced in the cell nucleus is measured as a function of time after radiation exposure. The disappearance of foci correlates with the repair of the DSBs. Recently, it has been found that the quality of the γ-H2AX foci, i.e. size and clustering, can be correlated with cell survival probability.8 However, standard microscopy does not provide sufficient resolution to characterize the quality of the foci. Microsphere-assisted imaging can be used in γ-H2AX assays to obtain enhanced images that overcome this limitation.
We stained U87 human glioblastoma (brain cancer) cells, irradiated by a clinical proton beam, with 4 ′ ,6-diamidino-2-phenylindole (DAPI) solution and γ-H2AX antibodies that reveal the location of the nuclei and nuclear foci formation, respectively. We then imaged them using a conventional microscope in fluorescence (FL) imaging mode, as shown in Figure 2(a). In Figure 2(b), the cell was imaged through a single 130μm-diameter BTG sphere attached to a glass coverslip by a PDMS layer with the microscope objective focused on the virtual image formed underneath the sphere. The red foci corresponding to DSBs cannot be resolved in Figure 2(c), which was obtained at the best imaging depth without using the sphere, but DSBs induced by the proton beam and manifested as red foci can be seen through the sphere in Figure 2(d). Comparing the images obtained without and with the microsphere shows the magnification and resolution advantages of microsphere-assisted imaging over conventional FL microscopy.
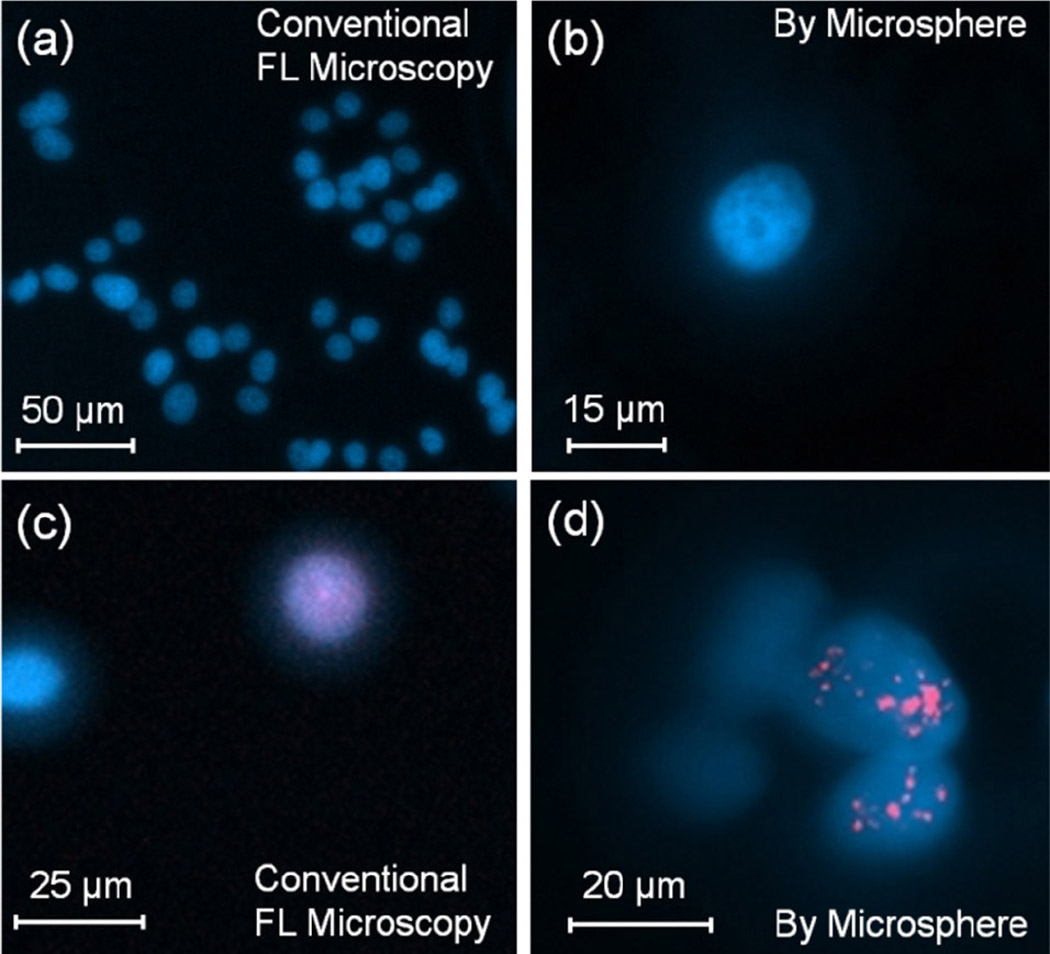
We also investigated using microsphere-assisted imaging for tissue sections. We imaged mouse kidney tissue sections to analyze immunostaining of glomeruli, which serve as the kidney's filtration units. Various glomerular diseases primarily target the glomerular filtration that controls the kidney's ability to filter blood.9 Diagnosing these conditions often requires histological analysis of kidney sections. A glomerulus is composed of three major cellular components: an endothelial (inner lining) layer, followed by the glomerular basement membrane, and podocytes (cells making up another layer) that together constitute the glomerular filtration assembly. Renal biologists screen the kidney sections for changes in the the cellular structures within a glomerulus, and they look for lesions and scarring in the glomeruli. This requires higher resolution than conventional microscopy provides, which is usually achieved through electron microscopy. We used FL microscopy and BTG microspheres (n∼2.1) immersed in a gold antifade mounting solution to image the distribution pattern of antibody-immunostained podocyte protein markers known as ZO-1 and Myo1c. It is known that these proteins are critical for maintaining podocyte structure and function, and their loss may severely affect kidney function. Thus, finding these proteins at the podocyte cell membrane indicates a healthy podocyte. Comparing the left and right panels in Figure 3 shows the improvements obtained by imaging through the microsphere. In the left panels, it is difficult to resolve the colocalization of Myo1c with ZO-1. However, in the right panels, captured through the microsphere, the colocalization of Myo1c with ZO-1 at the podocyte cell membrane within the glomerulus is clear.
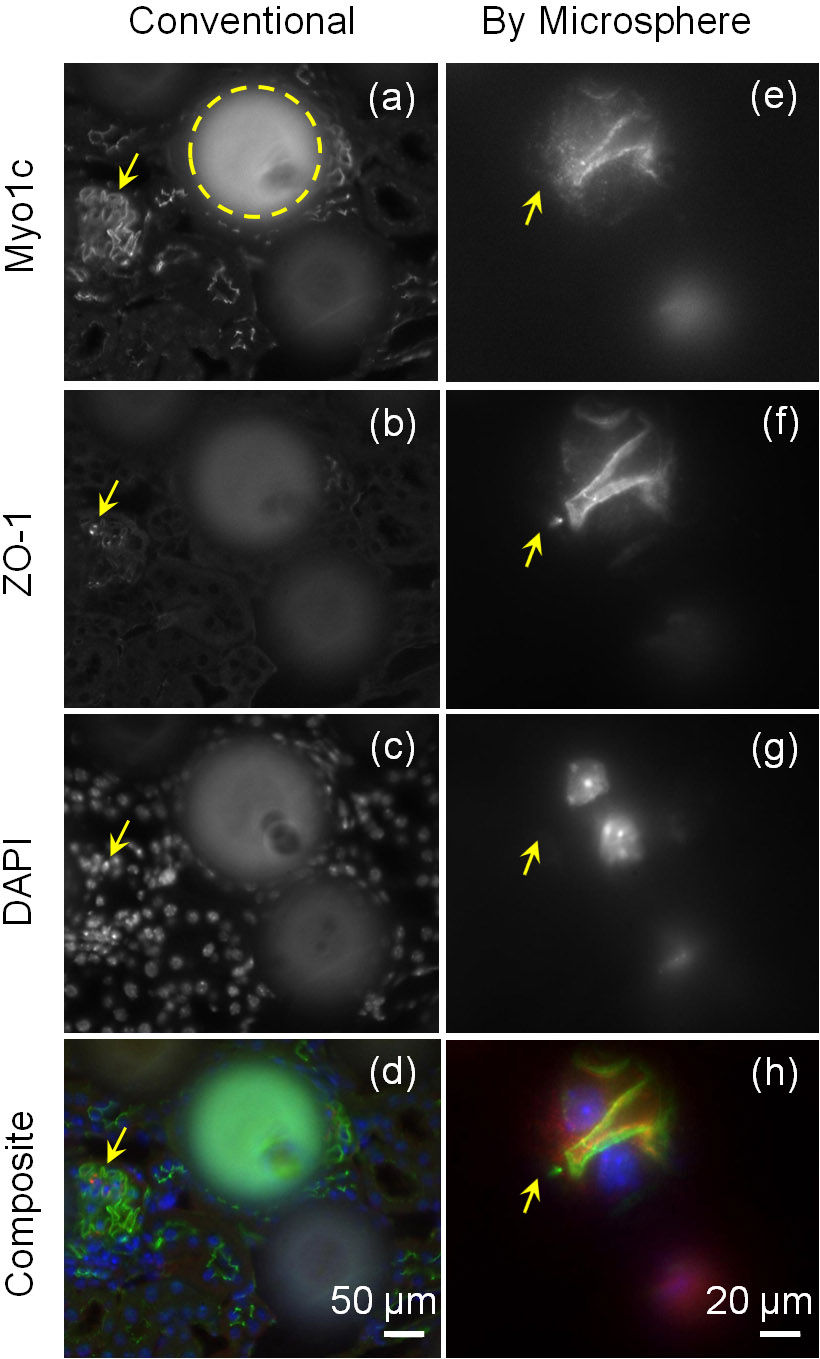
In summary, we have shown that microsphere-assisted super-resolution microscopy is feasible for examining biological and photonic structures. It uses slides composed of high-index microspheres fully or partially embedded in an elastomer layer. We are currently working on further advancing this technology, including optimizing the imaging capabilities and developing a large-scale fabrication technique. We are also expanding the application of microsphere-assisted imaging in cancer and medical sciences.
University of Pennsylvania
Arash Darafsheh pioneered the immersed microsphere-assisted super-resolution imaging technique. He holds an MSc in radiation medicine engineering, an MSc and PhD in optical science and engineering, and a postgraduate certificate in medical physics. He has published more than 50 papers, a patent, and a book chapter in the areas of optics and photonics, medical physics, and photodynamic therapy.
University of Pennsylvania