Visualizing laser-induced strain and fracture dynamics in crystals
Laser processing of various solids, specifically crystalline substrates, is an important part of manufacturing electronic devices such as computer chips, solar cells, dielectric substrates for thin films, and microelectromechanical systems.1, 2 Industrial laser users benefit by improving both the throughput and quality of the processing. Some crystalline substrates, however, are difficult to cut because lasers can easily generate cracks and dislocations in single crystals. For example, inside lithium fluoride (LiF) and magnesium oxide (MgO) single crystals, fractures are generated after irradiation with a tightly focused ultrafast laser pulse (see Figure 1).3 This damage changes the mechanical properties of the material, which changes its response to subsequent photoexcitation. Therefore, predicting where laser-induced fractures will form is important for uniform performance when scribing, cutting, or drilling crystalline substrates.
The laser processing community knows little about the short-term changes in materials as cracks and dislocations form, nor do we fully understand which forces induce them. To investigate further, our research team at Kyoto University recently observed strain dynamics in transparent solids using a time-resolved polarization microscope.4, 5 We chose LiF and MgO crystals as model cases to investigate strain and fracture dynamics because these crystals have mechanical properties that are simple to understand.
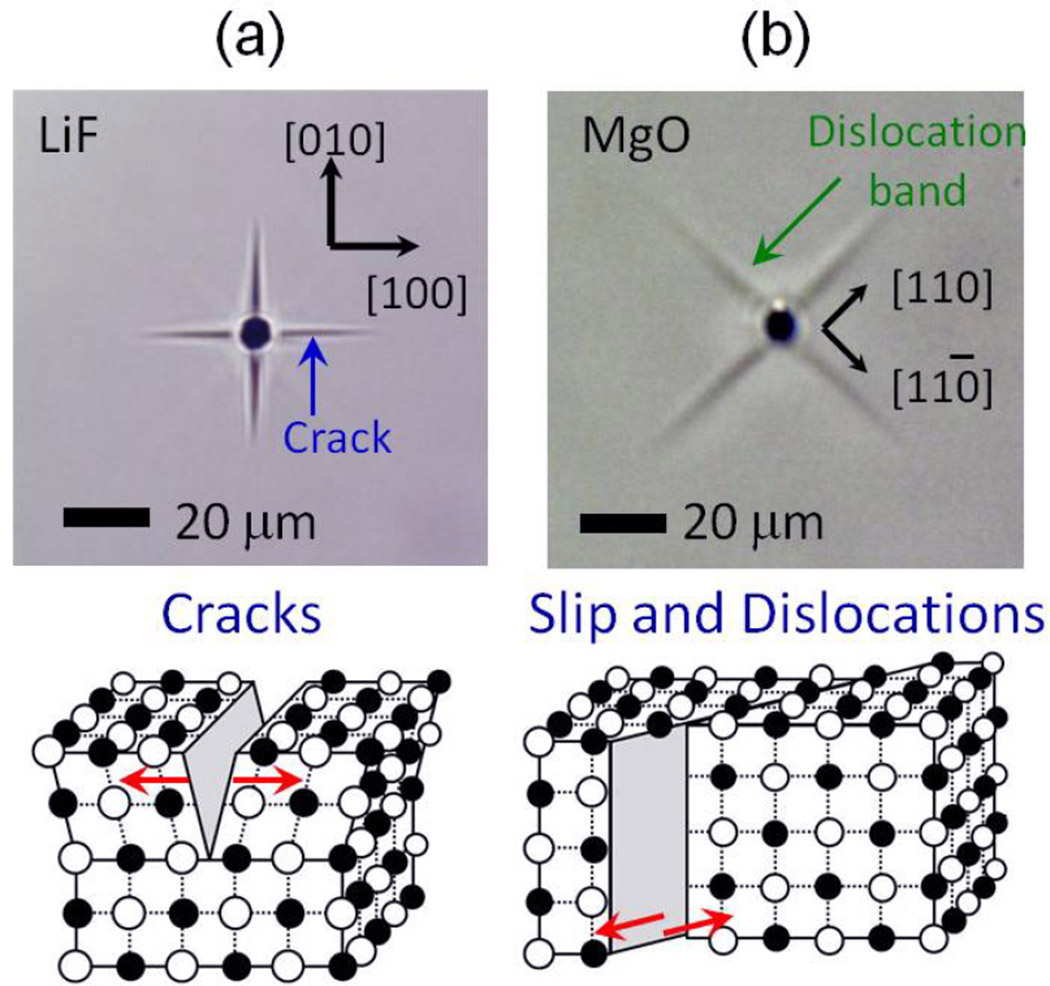
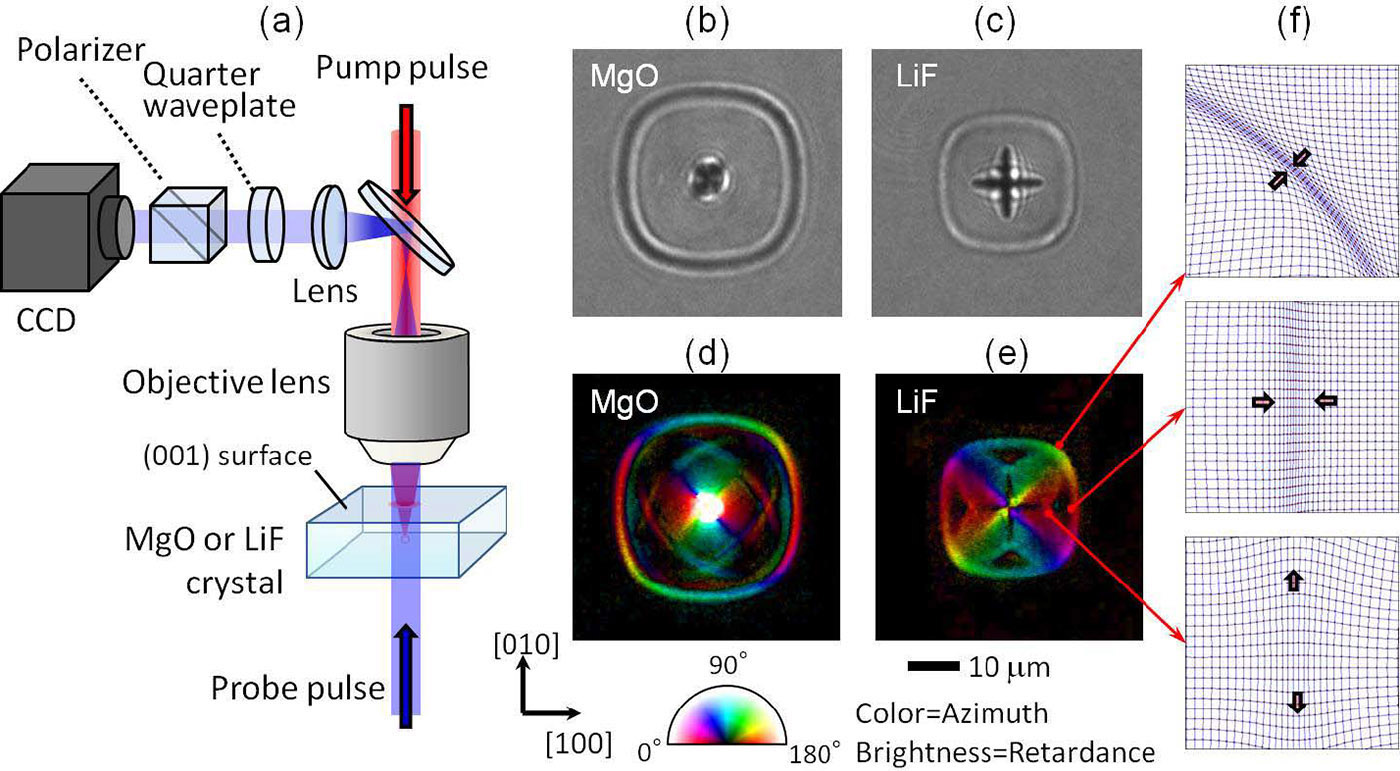
Our observation method, illustrated in Figure 2(a), uses a 100fs-long laser pulse at 800nm, tightly focused inside a sample, to induce nonlinear photoexcitation only in the focal volume. To obtain time-resolved images, we transmitted a probe pulse through the photoexcited volume from the opposite side. The 400nm probe pulse is a second-harmonic replica of the excitation pulse. In our setup, the transmitted probe light was imaged on a CCD camera. In addition, we placed a quarter waveplate and polarizer in front of the camera, which allows us to analyze polarization changes in the probe pulse. These changes in turn indicate the transient birefringence inside a sample induced by strain around the photoexcited region.
Time-resolved transmission optical microscopy images give us some information about the dynamics. In Figure 2(b), taken 3000ps after photoexcitation by a 2μJ ultrafast laser pulse, the central dark region in the MgO single crystal is the photoexcited material, and the slightly rounded square is a laser-induced stress wave. When we perform the same experiment on a LiF crystal, we observe a stress wave of a similar shape at the same time delay: see Figure 2(c). In addition, we observe the formation of cracks in the directions in the crystal. Although the transmission images show the dynamics of stress waves and crack generation, these images do not provide us with any information about strain or stress.
On the other hand, the birefringence images from the time-resolved polarization microscope—see Figure 2(d) and (e)—make visible the transient strain distribution. The birefringence images show not only the primary rounded-square stress wave but also more propagating regions of distributed strain. These secondary stress waves could be responsible for crack formation and propagation because they are located near the crack tips in the LiF crystal. According to our calculation of elastic dynamics, the strain in the secondary stress wave is normal to the propagation direction: see Figure 2(f). This means that the secondary stress wave is probably a major force that tears apart laser-induced cracks.
Stress amplitude can also be estimated from the birefringence images using elastic and photoelastic tensors of the crystals. For example, we estimate that the stress near the crack tip in a LiF crystal is about 1.5GPa at 1000ps, which is large enough to initiate a crack.
In summary, we observed the generation of stress waves and strain distribution after laser irradiation with an ultrafast pulse inside LiF and MgO single crystals using a time-resolved polarization microscope. These time-resolved observations give us a variety of ideas for improving laser machining techniques. Our next goal is to control laser-induced modifications, such as reducing crack generation, by modulating stress distribution. We may be able to achieve this by reducing the magnitude of the stress waves using interference. We have already found that the interference of stress waves disturbs crack propagation under some conditions and promotes it in others.6
Masaaki Sakakura is a program-specific associate professor in the Office of Society-Academia Collaboration for Innovation at Kyoto University. His research targets are the observation of dynamics in laser processing and development of novel laser processing techniques.
Yasuhiko Shimotsuma is an associate professor.
Kazuyuki Hirao is a professor.
Kiyotaka Miura is a professor.