Speeding light, mitigating loss: Hollow-core fibers step to the fore
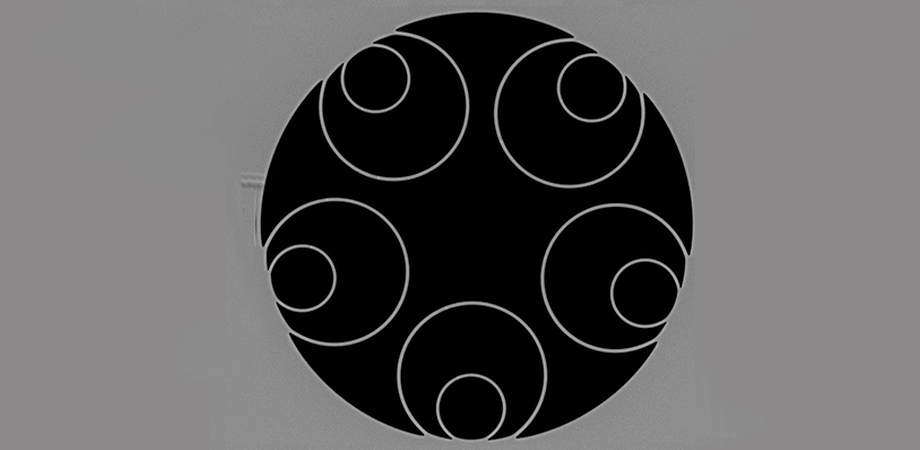
The trading floor of a stock exchange looked very different 30 years ago. Following the opening gong of the trading day, an observer would see a frenetic crowd of people, all on the telephone, hustling to buy and sell shares before the competition. Then, success could be measured in seconds. Today, when most trade is automated via algorithms, it’s measured in nanoseconds. Success no longer depends on having the fastest finger on the dial, but rather on the fastest optical fiber cable accessing the closest data center.
Several years of design improvements have brought hollow-core optical fibers close to the record for the world’s most transparent optical fiber. This year, the University of Southampton’s Optoelectronics Research Centre (ORC) reported a hollow-core fiber clearer than the best solid-core fibers in the 1,300-nm window. They also have demonstrated transmission of 1 kw of continuous laser power through a 1-km length of another hollow-core fiber.
The immediate allure of hollow-core fibers is that light travels through the air inside them at 300,000 km-per-second, 50 percent faster than the 200,000 km-per-second in solid glass, cutting latency in communications. Last year, euNetworks installed the world’s first commercial hollow-core cable from Lumenisity, a commercial spinoff of Southampton, to carry traffic to the London Stock Exchange. This year, Comcast installed a 40-km hybrid cable, including both hollow-core and solid-core fiber, in Philadelphia, the first in North America. Hollow-core fiber also looks good for delivering high laser power over longer distances for precision machining and other applications.
Hollow-core optical waveguides were first explored for laser communications 60 years ago, shortly after invention of the laser. With the best optical fibers at the time having loss of about 1,000 dB-per-km, Bell Labs expected hollow optical waveguides to be far more transparent—although at an inch or more in diameter, they were much larger than fibers.
But by 1972, Corning Glass Works had made solid silica fibers with attenuation of only 2 dB-per-km, and in 1978 Nippon Telegraph and Telephone had made fibers with attenuation below 0.2 dB-per-km. The current 0.1419 dB-per-km record for silica fibers at 1,560 nm was set in 2018 at a wavelength of 1,560 nm. Rayleigh scattering increases rapidly at shorter wavelengths, while infrared absorption of silica increases at longer wavelengths. Some materials may have lower absorption at longer wavelength, but none can match the durability of silica.
Research on hollow optical waveguides for 10-µm laser transmission began in the 1980s, but the revival of broader interest in hollow-core fibers began in the 1990s when Philip St. John Russell, director of the Max Planck Institute for the Science of Light, realized that arrays of subwavelength holes running along the length of an optical fiber could change the refractive index within the “holey” part of the fiber depending on the size of the holes. His group went on to arrange the holes to confine light of certain wavelengths in the hollow core in what was called a “photonic bandgap.” By 2005 they reported loss of only 1.2 dB-per-km at 1,620 nanometers in a hollow fiber, but that proved a plateau, and the low attenuation was limited to a narrow band of wavelengths less than 20-nm wide reflected by the photonic bandgap. Such fibers found some applications, but by the mid-2010s it was clear they would not widely displace solid-core fibers.
Dozens of other types of hollow-core and holey fibers were tested, but none of them could keep a broad range of wavelengths inside hollow core fibers without leaking out of the core into the higher-index glass cladding surrounding it. In the 1990s, theorists proposed creating an antiresonant layer at the core-cladding boundary that would keep light in the core from leaking into the cladding. Their idea was to create an antiresonant layer at the core-cladding boundary which would bounce light back into the hollow core rather than let it leak into the glass cladding, but it took a while to find a solution.
Deployment of a 7 km hollow-core fiber link between an Interexion data center and The London Stock Exchange using ultralow latency CoreSmart cable. Photo credit: euNetworks and Lumenisity
In 2014, ORC’s Francesco Poletti, a cofounder of Lumenisity proposed a way to create an antiresonant structure in a series of steps. The first was to run several parallel glass tubes along the fiber core, with one side touching the inside edge of the core-cladding boundary, and the other near the center of the core, to reflect much of the light back inside. Next, another tube would be nested into each of the tubes touching the core-cladding to further reduce how much light would leak out of the core into the cladding.
That wasn’t quite enough, however. If the nested tubes touched the other tubes on either side, some light could leak into the cladding through the touching point or node. Poletti thought separating the nested tubes so they did not touch their neighbors, would further reduce leakage out of the core, giving the hollow-fiber cable a very low attenuation. He called it a nodeless, antiresonant, nested hollow-core fiber (NANF).
These structures are complex, and not until 2017 did Poletti’s group demonstrate the first step, with nested tubes spaced around the core-cladding boundary of a 60-µm hollow core fiber, with light trapped in the central region between the nested tubes. That yielded a fiber with a loss of 10- to 20-dB-per-km across a range from 600 to 1,200 nm. Further refinements, including nodeless fibers with the nested tubes not touching each other, gradually brought loss down to 0.28 dB-per-km across the erbium-amplifier band from 1,530 to 1,620 nm, encompassing the wavelengths most widely used for long-distance wavelength-division multiplication.
This year, Poletti’s group reported another enhancement, a double-nested, antiresonant, nodeless fiber (DNANF). The hollow core contains five parallel tubes spaced evenly apart around the core-cladding boundary, not touching each other, and each of those tubes has two other tubes nested inside. They recorded a record low loss of 0.22 dB-per-km in the C band at 1,300 nm that beat anything achieved by solid-core fibers. The 0.174 dB-per-km at 1,560 nm was a record for hollow-core, but not solid-core fiber.
Poletti’s group is optimistic about further work. In a paper reporting their results they write: “After 30 years of research, [hollow-core fibers] HCFs have finally reached the loss level of solid core fibers. Analysis of the loss mechanisms of the prototype fiber reported here leads us to believe that DNANF technology still has margin for improvement. Further optimizations in fiber design and cabling procedures might one day yield cabled HCFs with a lower loss than possibly achievable in a conventional solid core optical fiber, whilst also benefiting from the other well-known advantages of latency, nonlinearity and dispersion in hollow core optical fibers.”
Like solid-core fibers half a century ago, the first major applications of hollow-core fibers are likely to be for communications. This time the stakes are highest for high-frequency securities traders who have complained for more than a decade—only partly tongue-in-cheek—that the speed of light is too slow to keep up with the market. After installing the world’s first hollow-core fiber communications cable last year, euNetworks followed this year with another cable 7-km-long to the London Stock Exchange.
5G wireless networks and cloud computing are also raising the stakes for latency in metro networks, cloud computing, the Internet of Things, and autonomous vehicles. Andrew Lord, senior manager of optical networks at BT (formerly British Telecom) says the ultralow nonlinearity of hollow-core fibers offers higher power transmission, and their wide bandwidth and low chromatic dispersion offer new transmission bands to carry more traffic. BT has tested hollow-core fiber in fronthaul for mobile and metro-core networks as well as for security training.
BT also is testing hollow-core for quantum communications: Its role would be to deliver the quantum keys that provide security for quantum encryption of communications. That’s a difficult problem because the quantum keys are transmitted as tens of photons, but the signals they encode include millions or billions of photons. The large number of signal photons would drown out the feeble quantum keys if the two were sent together. Researchers at ORC are working with colleagues at the University of Bristol, also in the UK, on ways to transmit the quantum keys through hollow-core fibers contained in the same cable as the separate solid-core fiber that would carry the higher bandwidth data signals.
Communications developers also are interested in the wide range of wavelengths—mostly shorter than 1,500 nm—where hollow-core fibers may be able to offer lower loss than solid-core fiber. So far, light-absorbing water has not been removed from hollow-core fibers, but Poletti says “if we can remove the water absorption, it could open up an extremely wide bandwidth” for wavelength-division multiplexing.
Recent experiments by Southampton show 1 kw of continuous near-diffraction-limited laser power at 1,070 nm through 1 km of hollow-core fiber. Transmission of that much power in solid-core fibers is limited to tens of meters. What’s important in delivering that power is not the attenuation of 0.74 dB-per-km, but the reduction of nonlinear effects by three orders of magnitude arising from the way in which the hollow-core fiber guides the beam. The core region where the NANF concentrates light is 31 µm wide, too large to limit propagation to a single mode. However, higher-order modes launched into the hollow core would quickly leak out of the core into the surrounding glass and be lost, leaving an essentially pure singlemode beam that can travel through 1 km of the hollow core fiber. Longer transmission distances and higher power levels could benefit laser machining.
Keeping the light out of glass also reduces dispersion in hollow-core fibers to about a tenth of that in solid-core fibers. Low dispersion is not important in coherent long-haul communications because electronic compensation corrects for dispersion and other flaws. However, other applications may benefit from the low dispersion of hollow-core fiber.
The recent advances in hollow- core fibers have been impressive and the range of applications are promising, but important challenges remain. The ecosystem for manufacturing and installation is still immature so “interest is likely to remain in specialist, high-value applications for some time,” says Sergei Markovejs of Corning Optical Communications.
Some concerns remain for hollow-core fibers, but progress is already being reported on improving light-coupling into them. Carmelo Scarcella of CERN in Geneva, Switzerland, has tested NANF for planned improvements to the Large Hadron Collider. Tens of thousands of point-to-point links now transmit tens of Tbits-per-second of data over radiation-hardened solid-core fiber. To test hollow-core fiber, they coupled 25 Gbit-per-second signals from silicon photonics circuits through a 50-m length of NANF. They observed excess loss in the hollow-core fibers but said that could be overcome by designing grating couplers for the task, paving the way to take advantage of hollow-core fiber for its high radiation tolerance. Solid-core fiber will not go away soon. It’s a well- established technology, easy and inexpensive to manufacture, and durable in the field. But hollow-core fiber is definitely starting to find its own niches.
Jeff Hecht is an SPIE Member and freelancer who writes about science and technology.
Enjoy this article? Get similar news in your inbox |
|